Metabolic MRI
Metabolic information from the human brain, body and skeletal muscle can be obtained by in vivo magnetic resonance spectroscopy (MRS), magnetic resonance spectroscopic imaging (MRSI), non-proton MRI and MRS, as well as chemical exchange saturation transfer (CEST) imaging. While most of these methods use endogenous contrasts, some need simplistic tracers such as glucose.
1H MRS & MRSI
Non-invasive methods including proton magnetic resonance spectroscopy (1H MRS) and spectroscopic imaging (1H MRSI) allow for the detection of about 20 metabolites and compounds in the brain and body without need of contrast agents. It provides rich information about energy metabolism, neurotransmission, myelination, cell membrane metabolism, osmoregulation, antioxidant profile, and protein synthesis. 1H MRS can be applied for both human and preclinical studies. The investigation of steady state mean tissue concentrations as well as their dynamic changes is possible.

Contact PIs: Anke Henning, Kimberly Chan, Manoj Sarma
31P MRS & MRSI
Phosphorous magnetic resonance spectroscopy (31P MRS) and spectroscopic imaging (31P MRSI) are non-invasive methods that detect about 10 metabolites and compounds in the brain and body without the need for contrast agents. This provides rich metabolic information such as intracellular and extracellular pH, magnesium concentrations and metabolic turnover rates. The latter becomes possible via saturation transfer experiments and in bore exercise. 31P MRS can be applied for human as well as preclinical studies. The investigation of steady state mean tissue concentrations as well as their dynamic changes is possible.

Contact PIs: Jimin Ren, Anke Henning
13C MRS & MRSI
Another non-invasive method, carbon magnetic resonance spectroscopy (13C MRS) enables detection and detailed characterization of fatty acids and glycogen, based on the 1% natural abundance of the MRI detectable isotope 13C. Furthermore, we can observe the enrichment of 13C labelling in about 10 additional metabolites and compounds in the brain and body after intake of 13C glucose. Respective enrichment time courses obtained by time-resolved experiments make it possible to calculate in vivo metabolic turnover rates including glycolytic, Krebs cycle, and neurotransmitter cycle rates. 13C MRS can be applied for human as well as preclinical studies.
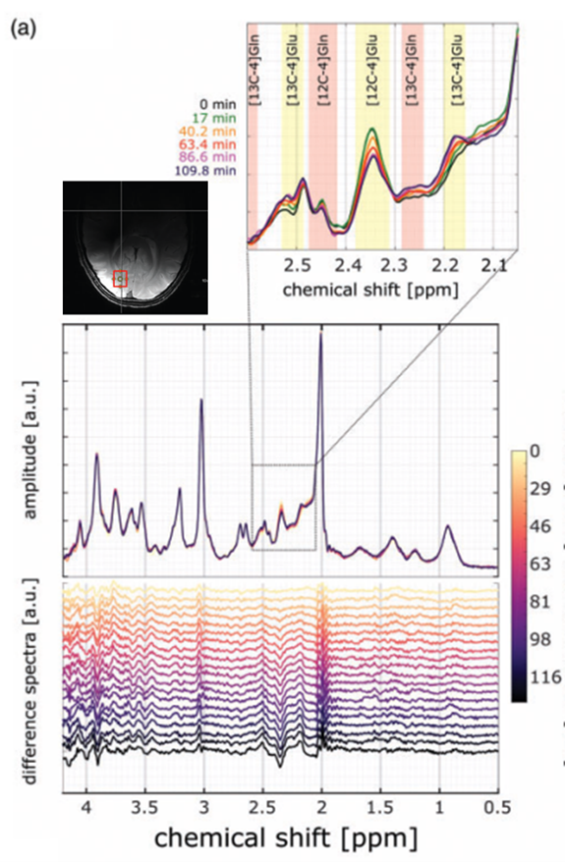
Contact PIs: Anke Henning, Ivan Dimitrov
2H MRS & MRSI
The non-invasive Deuterium magnetic resonance spectroscopy (2H MRS) method allows researchers to detect heavy water and deuterated fatty acids based on the 0.015% natural abundance of the MRI detectable isotope 2H. The enrichment of 2H labelling can be observed in glucose, glutamate, and lactate in the brain and body after intake of 2H-labelled glucose. Respective enrichment time courses obtained by time-resolved experiments enable the calculation of in vivo metabolic turnover rates including the glycolytic rate and the Krebs cycle rate. 2H MRS can be applied for human as well as preclinical studies.
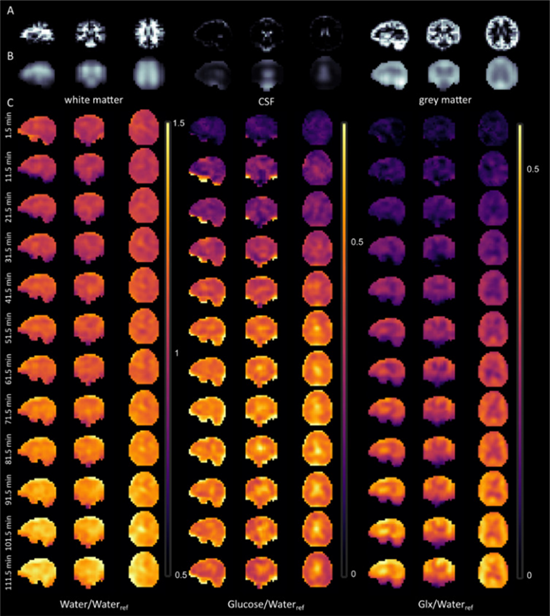
Contact PI: Anke Henning
23Na MRI
Sodium MRI detects endogenous sodium ion concentrations in the brain, cartilage, or body non-invasively. Sodium, the second most abundant element in vivo, remains crucial to maintain osmoregulation and cell physiology. Sodium affects the energy-consuming processes of membrane transport and neurotransmission, plus it acts as a counter ion for balancing charges of tissue anionic macromolecules.
Contact PIs: Anke Henning, Ivan Dimitrov
Chemical Exchange Saturation Transfer (CEST)
Chemical Exchange saturation transfer imaging measures the exchange of protons between macromolecules and tissue water. Thus, the tissue macromolecular content can be non-invasively determined. A specific focus includes detecting proteins via the amide proton transfer (APT) contrast. In addition, CEST imaging allows for measuring intermolecular interactions via the Nuclear Overhauser effect (NOE), chemical exchange rates, metabolite content, and pH.
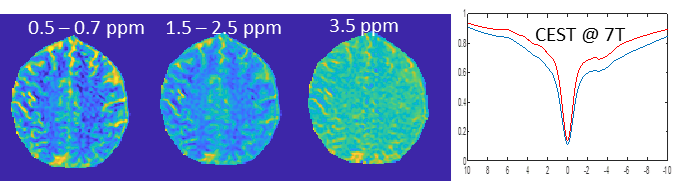
Contact PIs: Anke Henning, Dean Sherry
Novel Data Acquisition Methods
MR spectra can be obtained from a single localized volume of interest, or metabolic images can be derived from spectroscopic imaging. Robust localization, encoding, and artefact suppression methods are developed to ensure optimal data quality. In addition, edited and 2D spectroscopy techniques are implemented to reduce spectral overlap and to increase the number of detectable metabolites. The information encoded into CEST data on the other hand becomes very sensitive to the applied RF field strength. Hence, RF shimming is evaluated for CEST. All metabolic MRI acquisition methods are intrinsically slow due to the need to encode and resolve the metabolic information paired with a low signal-to-noise ratio. Acceleration of the data acquisition while conserving signal strength remains another important research focus.
Contact PIs: Anke Henning, Kimberly Chan, Manoj Kumar Sarma
Novel Data Analysis Methods
Preprocessing, image reconstruction, and quantification pipelines are important for accurate and precise metabolic readouts. The development of comprehensive single voxel and spectroscopic imaging reconstruction and processing pipelines accompanies our own spectral fitting software and quantification pipelines for proton and non-proton MR spectroscopy. Highlights of these developments include a neural-network-based spectroscopic imaging reconstruction method and the spectral fitting package that outperforms the gold standard in the field. In addition, researchers create novel spectral models for macromolecules, downfield spectra, and labelled isotope data. They also attempt spatially resolved metabolic modelling.
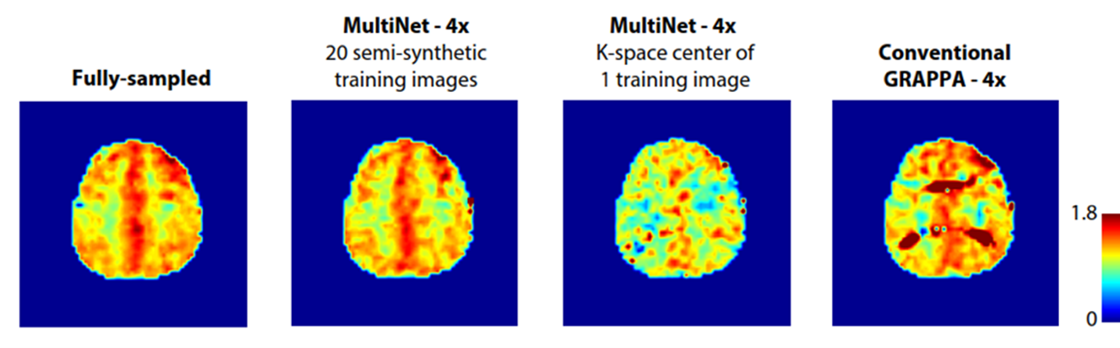
Contact PIs: Anke Henning, Kimberly Chan
Applications and Clinical Translations
Metabolic MRI methods can determine mean tissue concentrations of metabolites and compounds along with pH, ion concentrations, and metabolic turnover rates. In addition, these methods help evaluate the change of all these parameters over time upon disease progression, treatment response, functional tasks, exercise or 13C or 2H label intake. Therefore, researchers use metabolic MRI methods for clinical diagnostics, as well as neuroscientific, physiological, and clinical trials. These studies generally apply to the human brain and organs including breast, spinal cord, liver, kidney, prostate, and heart, as well as the skeletal muscle and adipose tissue.
Examples for current studies are given in the sections:
Contact PIs: Jimin Ren, Anke Henning, Kimberly Chan, Manoj Sarma