UT Southwestern nearly doubles lifespan of mice with brain cancer
New technology creates vastly better drug delivery system
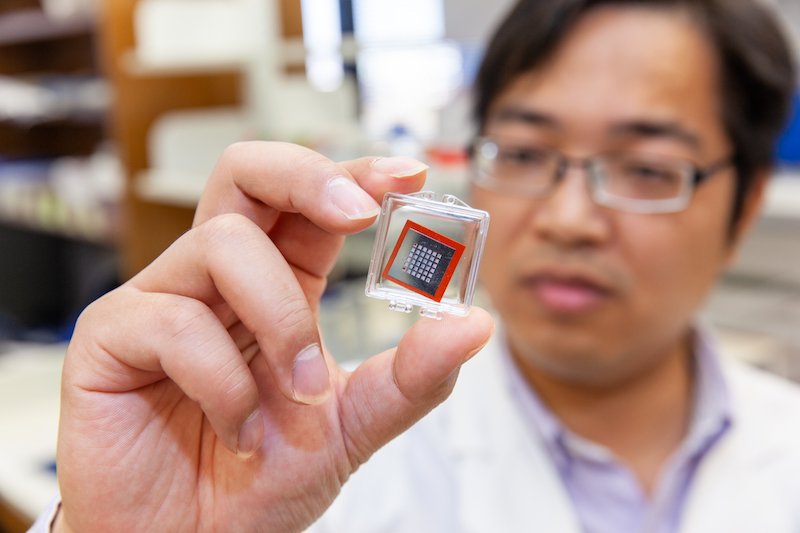
DALLAS – Feb. 6, 2020 – UT Southwestern Simmons Cancer Center researchers have developed technology that is spawning a much better form of drug delivery for gene therapy and has achieved a 43 percent increase in the survival of mice with glioblastoma, one of the deadliest forms of human brain cancer. The researchers used specially engineered silicon chips to zap cells and produce a new drug delivery system. The results were published in Nature Biomedical Engineering.
“We were shocked at our findings. We have opened a new door for gene therapy,” says Zhaogang Yang, Ph.D., assistant professor of radiation oncology at UT Southwestern Medical Center and the lead author of the study. He says his team believes it is the best drug delivery for gene therapy seen in a lab to date. It appears that it can be used in gene therapy drug delivery in any type of cancer and in other diseases such as genetic disease and cardiovascular disease.
The Cellular NanoPorotation Chips were designed with a million tiny holes in them and were originally intended to deliver biological cargo into skin cells in mice. The skin tests worked, so Yang decided to try using the dime-sized chips to get DNA into mouse cells with a gene that is often mutated in the brain cancer, glioblastoma.
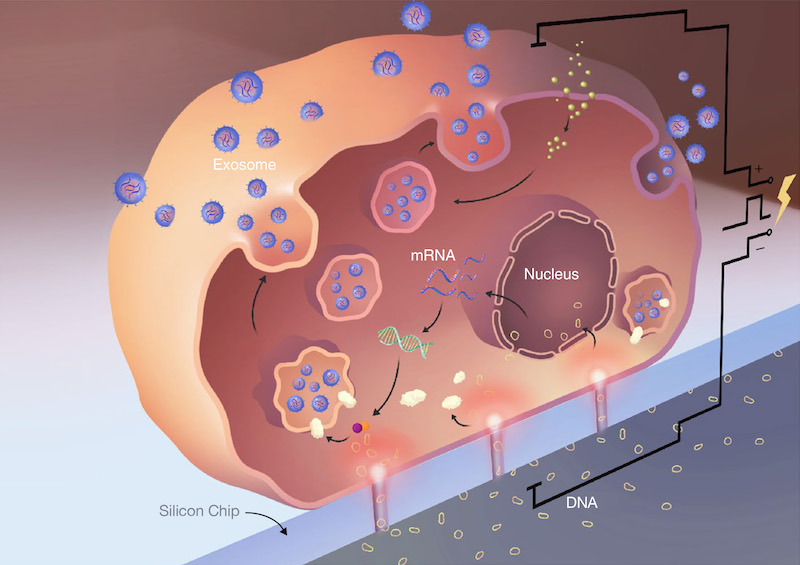
Yang’s team placed mouse cells on one side of the chip and on the other side had small circles of DNA containing PTEN, a gene that suppresses tumor growth. Then they zapped the chips with about the same voltage of a lightbulb. The cells’ natural response to brief electrical shocks caused the membranes to expand momentarily, opening tiny pores that gave scientists an opportunity to smuggle DNA into the cell.
Twenty-four hours after the cells were zapped they were found to carry PTEN. But to the scientists’ surprise, they also found that the cells, while recovering from the electrical shock, had spit out exosomes, tiny membrane-encased bubbles that can travel through the bloodstream. When the team examined the exosomes they found them to have 10,000 times more PTEN mRNA than other mRNA-production techniques. Oncologists prize mRNA for the important role it plays in reintroducing PTEN into cancer cells.
Using lasers to measure the quantity of exosomes, the team found that the cells had generated about a million exosomes – 50 times more exosomes than standard exosome-generating techniques. The exosomes and their abundance were a great find, because the scientists believed the exosomes could travel through the bloodstream and deliver PTEN directly to the cancer cell.
“This innovation gives targeted gene therapy a badly needed advance in drug delivery,” says Carlos L. Arteaga, M.D., director of the Harold C. Simmons Comprehensive Cancer Center. “Electricity defined the first half of the 20th century, and silicon chips defined the second half. This innovation combines electricity and silicon chips to break new ground in drug delivery.”
The team collected the exosomes containing PTEN and injected them into the tails of 20 mice with human glioblastoma. Mice with the exosomes produced by the Cellular NanoPorotation Chip lived 70 days. Mice with standard treatment lived 40 days.
Using a PTEN gene that generates a PTEN protein that glows, the researchers were able to look at mouse brains and confirm that PTEN had found its way to the tumor. It was proof that the exosomes produced by the Cellular NanoPorotation Chip had traversed the blood-brain barrier, one of the most difficult hurdles in the body. This was evidence that it could go anywhere in the body, according to Wen Jiang, M.D., Ph.D., an assistant professor of radiation oncology and member of the Simmons Cancer Center who was also part of the study.
“If we can encapsulate large amounts of mRNA into these exomes and deliver them into the right place in an efficient manner, it will open up a whole host of potential areas for genetic disease, autoimmune disease, cardiovascular disease and inborn errors of metabolism in addition to cancer,” Jiang says.
According to Yang, each silicon chip costs less than $10 and can be used at least 50 times.
Yang has moved on to testing whether the procedure can produce exosomes with PTEN combined with other genes implicated in cancer.
Additional study authors are Junfeng Shi of the Department of Chemical and Biomolecular Engineering, The Ohio State University, Columbus, Ohio, Jing Xie, School of Life Sciences, Jilin University, Changchun, China, Yifan Wang of the Department of Radiation Oncology, UT Southwestern Medical Center, Jingyao Sun of the Department of Chemical and Biomolecular Engineering, The Ohio State University, Tongzheng Liu of the College of Pharmacy, Jinan University Guangzhou, China, Yarong Zhao, School of Life Sciences, Jilin University, Changchun, China, Xiuting Zhao, School of Life Sciences, Jilin University, Changchun, China, Xinmei Wang, of the Department of Chemical and Biomolecular Engineering, The Ohio State University, Yifan Ma of the Department of Chemical and Biomolecular Engineering, The Ohio State University, Veysi Malkoc of the Department of Chemical and Biomolecular Engineering, The Ohio State University, Chiling Chiang of the College of Medicine, The Ohio State University, Weiye Deng of the Department of Radiation Oncology, UT Southwestern Medical Center, Yuanxin Chen of the Department of Neurological Surgery, Mayo Clinic, Jacksonville, Florida, Yuan Fu the Department of Neurological Surgery, Mayo Clinic, Jacksonville, Florida, Kwang J. Kwak of the Department of Chemical and Biomolecular Engineering, The Ohio State University, Yamin Fan of the Department of Chemical and Biomolecular Engineering, The Ohio State University, Chen Kang of Carver College of Medicine, University of Iowa, Iowa City, Iowa, Changcheng Yin of the Department of Biophysics, Health Science Center, Peking University, Beijing, China, June Rhee of Institute for Stem Cell Biology, Stanford University, Palo Alto, California, Paul Bertani of the Department of Electrical and Computer Engineering, The Ohio State University, Jose Otero of the Department of Neuroscience, The Ohio State University, Wu Lu of the Department of Electrical and Computer Engineering, The Ohio State University, Kyuson Yun of Peak Brain and Pituitary Tumor Center, of the Department of Neurosurgery, Houston Methodist Research institute, Weill-Cornell Medical College, Houston, Texas, Andrew S. Lee of Institute for Stem Cell Biology, Stanford University, Palo Alto, California, Lesheng Teng of School of Life Sciences, Jilin University, Changchun, China, Betty Y. S. Kim of the Department of Neurosurgery, UT MD Anderson Cancer Center, Houston, Texas, and L. James Lee of the Department of Chemical and Biomolecular Engineering, The Ohio State University.
The authors declared no competing interests.
About UT Southwestern Medical Center
UT Southwestern, one of the premier academic medical centers in the nation, integrates pioneering biomedical research with exceptional clinical care and education. The institution’s faculty has received six Nobel Prizes, and includes 22 members of the National Academy of Sciences, 17 members of the National Academy of Medicine, and 14 Howard Hughes Medical Institute Investigators. The full-time faculty of more than 2,500 is responsible for groundbreaking medical advances and is committed to translating science-driven research quickly to new clinical treatments. UT Southwestern physicians provide care in about 80 specialties to more than 105,000 hospitalized patients, nearly 370,000 emergency room cases, and oversee approximately 3 million outpatient visits a year.