A sharper focus
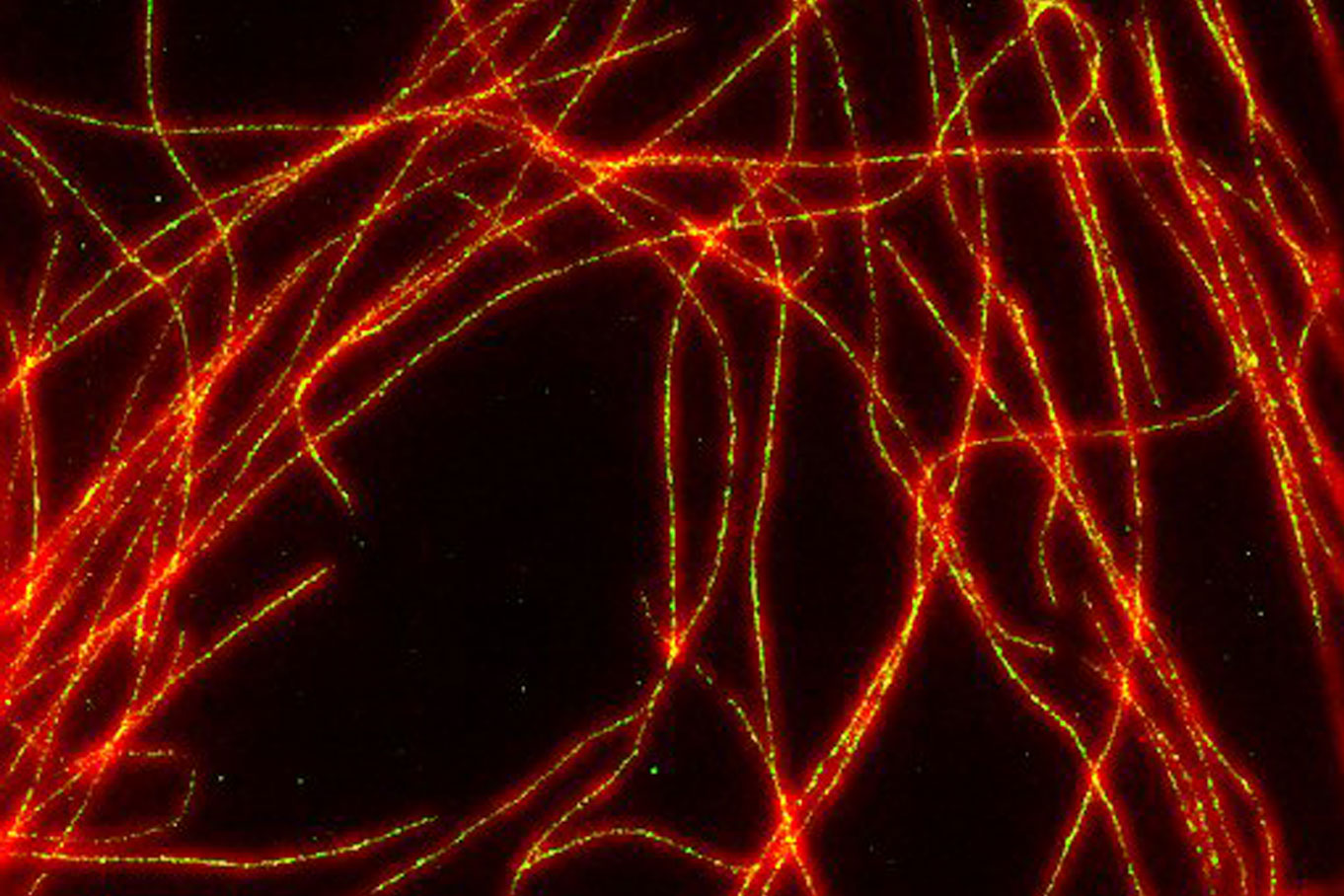
The 16th-century spectacle makers who invented the microscope would be amazed by what researchers at UT Southwestern and elsewhere can now do with the progeny of their creation.
On a recent afternoon, Dr. Nan Yan, Associate Professor of Immunology and Microbiology, sat at a spinning disk confocal microscope here, watching a protein make its way across a living cell taken from a mouse. In another room in the University’s Live Cell Imaging Core Facility, a small group of researchers huddled around a confocal laser scanning microscope to examine collagen surrounding a tumor in a zebrafish embryo.
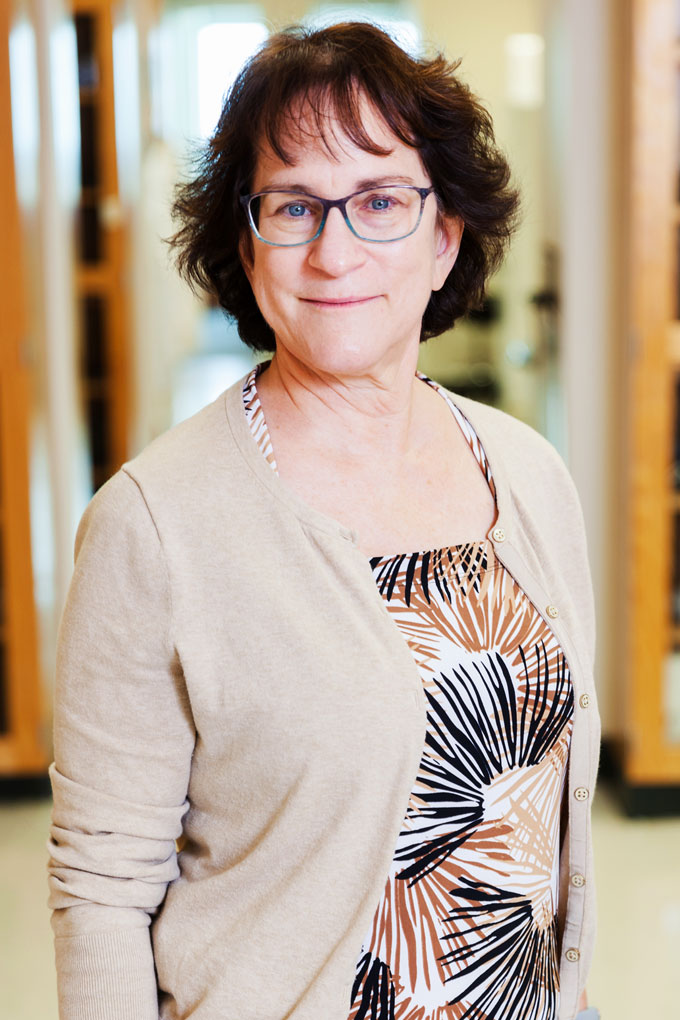
“It’s amazing what we can see now,” says Dr. Katherine Luby-Phelps, Director of the Facility that houses 11 high-powered optical, or light, microscopes. Instead of magnifying lenses, a lightbulb, and an eyepiece to look through, these instruments come with laser beams, cameras, and computers that can capture digital images so researchers can peer inside life itself.
Unlike their more basic predecessors – which can still be bought for a few hundred dollars – the most advanced microscopes today easily run half a million dollars, Dr. Luby-Phelps says. “Some of my microscopes cost a million,” she adds, which is well beyond the reach of most individual researchers and even smaller university departments. The Live Cell Imaging Core, which opened in 2004, provides access to this sophisticated technology for a modest fee.
The Core rents use of the instruments by the hour to campus researchers, charging between $15 and $50, depending on the microscope, and also lets researchers from other universities and industry use them (albeit at a higher hourly rate).
Almost 300 researchers from more than 150 UT Southwestern laboratories use the Facility in a typical year, says Dr. Luby-Phelps, resulting in more than 400 peer-reviewed articles since 2004.
Improving technology
Although electron microscopes, developed in the 1930s, can image objects as small as atoms – smaller than an optical microscope’s reach – those microscopes come with an important disadvantage. “To use electron microscopy, you have to kill the specimens. The sample chamber is under high vacuum and the electron beam just fries them,” says Dr. Luby-Phelps, who is also a Professor of Cell Biology and Director of the Electron Microscopy Core Facility, housed on a different floor in the same UTSW building.
“These instruments come with laser beams, cameras, and computers that can capture digital images so researchers can peer inside life itself.”
Optical microscopes, on the other hand, allow researchers to peer inside a living cell.
And optical microscopes are rapidly improving. “Since the 1990s, there has been an explosion in the technology in light microscopy,” Dr. Luby-Phelps says.
“Light microscopy is undergoing a massive revolution,” adds Dr. Gaudenz Danuser, founding Chair of the Lyda Hill Department of Bioinformatics and Professor of Bioinformatics and Cell Biology. “Thanks to super-resolution, it has reached the single nanometer scale, and at the same time more and more optical tricks are being invented to make light go deep into tissues.
“We are not far from being able to acquire information about every single molecule in a full tissue sample – that is a scaling factor of a trillion – and all this in three dimensions over time. Every other biomedical data type is dwarfed by that,” he says.
Optical phenomenon
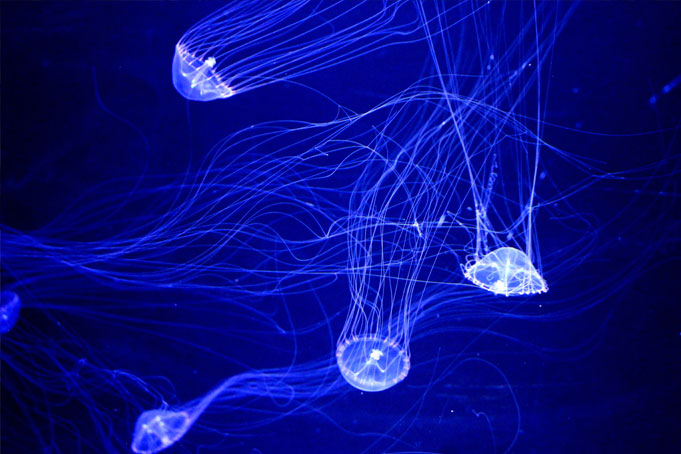
A 1960s finding by a researcher working with fluorescent jellyfish off the coast of Washington led to this transformation. Dr. Osamu Shimomura discovered that jellyfish contain a green fluorescent protein that glows when hit with ultraviolet or blue light.
It wasn’t until the 1990s that other researchers figured out how to clone the gene responsible for the jellyfish’s fluorescence, create additional colors, and use these fluorescent tags to track movement of biological parts within living cells under a microscope.
Microscope makers responded by refining their instruments, halving the size at which objects can be clearly seen and differentiated, says Dr. Luby-Phelps. (Historically, precise imaging was limited by the wavelength of visible light to about 250 nanometers, she says.) More recent advances have pushed the visual limit down further still, closer to a single nanometer under optimal conditions, she says.
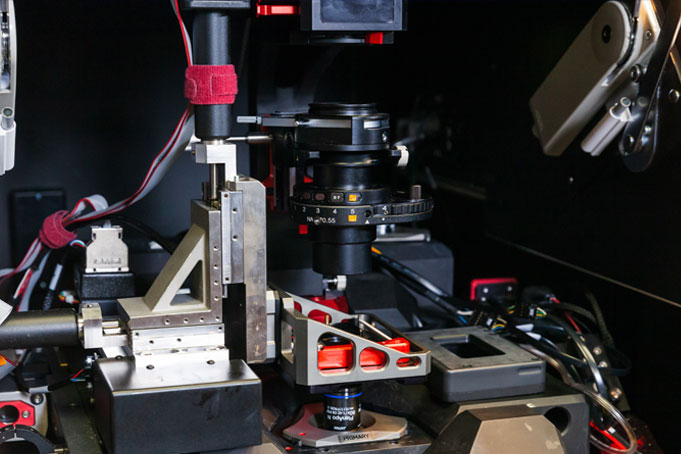
The Core Facility’s most advanced microscope, the OMX-SR Super-Resolution, can bring targets 120 nanometers in size into focus – 20 nanometers in some modes, she says. It does that by beaming light from different angles, then sending the information to a computer to reconstruct the image, she explains.
This is the microscope UT Southwestern researchers used to determine how circulating LDL cholesterol makes its journey across the cells lining arteries to enter the artery wall, where it drives the development of atherosclerosis. Dr. Philip Shaul, Director of the Center for Pulmonary and Vascular Biology, Vice Chair of Research, and Professor of Pediatrics, reported this research in a Nature article published in April.
“Dr. Luby-Phelps and the Live Cell Imaging Core Facility played a key role in our studies determining how LDL cholesterol moves from the circulation across the endothelial cells that line blood vessels,” says Dr. Shaul.
3D imaging capabilities
The Core Facility also has four confocal laser scanning microscopes, which scan a specimen one depth level at a time, then feed the information into a computer to create a clear 3D reconstruction without the blur caused by input from parts of the specimen that are not in focus.
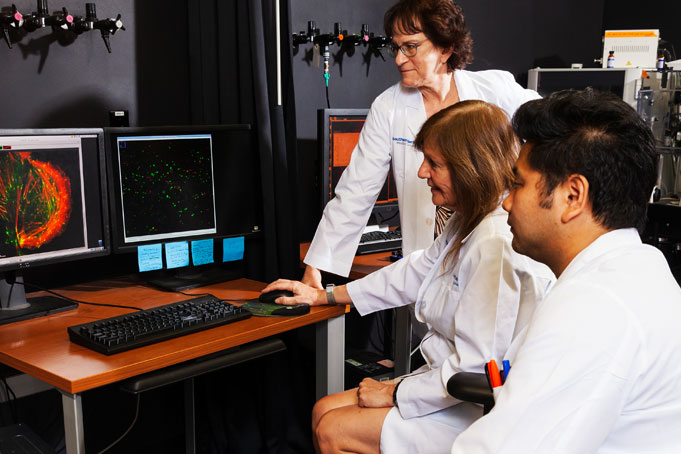
Two wide-field deconvolution microscopes, designed to expose the entire thickness of the specimen to light at the same time, are also available. This process doesn’t give as sharp an image, since out-of-focus layers will be included, Dr. Luby-Phelps says. But the snapshot approach is faster and useful when a researcher needs to capture something happening quickly within a cell, she says.
This is the kind of expert information and advice the Core Facility’s staff offers along with access to the microscopes.
For researchers, both are important, Dr. Danuser says. “It is impossible for a single lab to keep up with the diverse modes of imaging that are being developed every day,” he says. “On the other hand, it is clearly expected these days that biomedical studies use state-of-the-art techniques. A core facility like this plays an absolutely central role on our path to scientific discovery.”
Dr. Danuser holds the Patrick E. Haggerty Distinguished Chair in Basic Biomedical Science.
Dr. Shaul holds the Associates First Capital Corporation Distinguished Chair in Pediatrics.
Dr. Yan is a Rita C. and William P. Clements, Jr. Scholar in Medical Research.